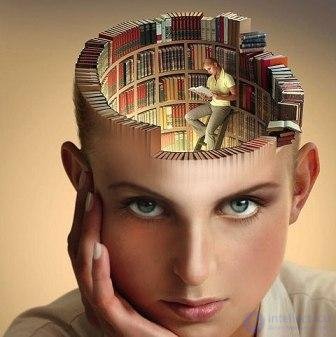
This series of articles describes a wave model of the brain that is seriously different from traditional models. I strongly recommend that those who have just joined begin reading from the first part.
In the previous section, we showed how distributed memory can look. The basic idea is that a common wave identifier can unite neurons, which by their activity form a memorized picture. To reproduce a specific event, just run the corresponding memory identifier on the cortex. Its distribution will restore the same picture of activity that was on the cortex at the time of fixation of this memory. But the main question is how do we get the required id? The associativity of memory implies that, by the set of features, we can select events in the description of which these signs were present. That is, there must be a neural mechanism that allows, by the description in certain attributes, to get an identifier suitable for these attributes of memory.
When we talked about the propagation of neural waves, we proceeded from the fact that the neuron stores on the extrasynaptic membrane those wave patterns, of which it is a participant. Having met a familiar picture, the neuron with its spike creates a continuation of the unique pattern. And here it is important that the neuron is not just able to recognize the wave pattern, but the fact that he himself is part of a propagating pattern. Only by being part of a unique wave is a neuron able to participate in its distribution.
In order not to get confused in the subsequent reasoning, we repeat the basic properties of the wave model of the cortex. If we mark the neurons belonging to the same wave pattern or - in other words - the identifier, then we get something like a set of points shown in the figure below.
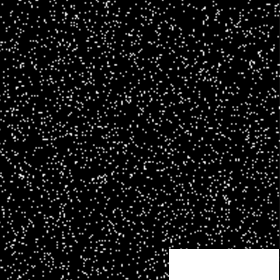
By taking any place of the cortex and activating the elements of the identifier, we will get a wave propagating from the active place, repeating the pattern characteristic of the identifier (figure below).
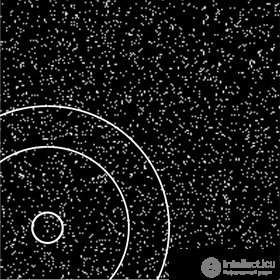
Passing through each place of the cortex, the wave will “highlight” a fragment of its unique pattern. So, having started from area 1, the wave, having reached area 2, will create there a unique pattern predetermined by the identifier (figure below).
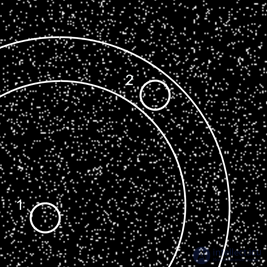
By the uniqueness of the pattern, it is possible to determine in each place of the cortex which identifiers make up the wave.
If we reproduce the pattern already familiar to us on a quiet bark in area 2, then it will also create a wave that, having spread to area 1, will create there the same pattern characteristic of this identifier.
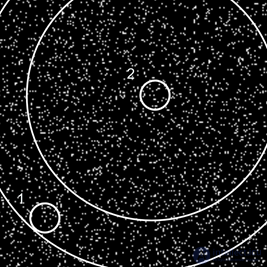
From all this it follows that for identifying the identifier it is enough to remember at any place in the cortex which pattern in this place is created by the wave. This can be memorized either at the neuron synapses, or on the extra-synaptic part of the membrane. Memorization at synapses leads to evoked activity (a packet of impulses) during recognition, memorization on metabotropic receptive clusters allows to obtain single spikes at the appearance of a familiar wave.
More difficult if we need to reproduce the identifier. To do this, we need to activate, at least in single spikes, a group of closely located neurons related to the required identifier. Describing the reverse projection and introducing several mediators, we just showed a mechanism that implements something similar.
Speaking about the associativity of concepts, we have shown that a query built on waves of identifiers returns a set of identifiers of concepts associated with the concepts contained in the query.
In order to show the mechanism for extracting memories from associative event memory, we need to show how a wave query consisting of notions-attributes can produce unique hippocampal identifiers of memories suitable for this query. If we can get such a set of identifiers and select one of them, then by running this identifier back along the cortex, we get the activity of neuron detectors containing this identifier on the extrasynaptic membrane, which would be equivalent to restoring the entire descriptive picture of the required memory.
Let me remind you about our simplified and schematic description. Next, I will outline a possible neural mechanism of associative memory, without claiming that the brain works that way.
Again, briefly turn to the structure of real neurons. The body of the neuron, the soma, has a limited area and cannot provide a place for all synaptic contacts. Most synapses fall into a branched structure called a dendrite or dendritic tree (figure below). The number of synapses located on the dendrite is 10-20 times higher than the number of synapses on the soma.
Forms of dendritic trees (Greg Stuart, Nelson Spruston, Michael Häusser) It was found that a neuron behaves differently depending on whether signals arrive at synapses on one or different dendritic branches (Shepherd GM, Brayton RK, Miller JP, Segev I., Rinzel J., Rall W., 1985 ). The simultaneous arrival of pulses at the synapses of one branch causes a much stronger neuron response than a signal distributed across different branches.
Based on observations of this kind, the hypothesis was born that dendritic branches can play the role of coincidence detectors (Softky, 1994). Its essence is that the signals generated in the remote branches of the dendritic tree need to generate segments of the dendritic tree along the signal path to the soma to generate neuron spikes.
This effect was shown for pyramidal neurons (Jarsky T., Alex Roxin A., Kath WL, Spruston N., 2005), but it can be assumed that something similar is characteristic of other types of neurons.
Phases of signal propagation in the apical and oblique segments of the pyramidal neuron of the hippocampus. The signal arises in the apical bundle (red dot) and propagates to the Schaffer collateral (green dot). The signal in the place of the dendrite closer to the catfish does not allow the distant signal to fade and contributes to the spike (Jarsky T., Alex Roxin A., Kath WL, Spruston N., 2005) The propagation of a pulse along a dendrite is accompanied by its considerable attenuation. In theory, the influence of distant (distal) synapses should be significantly less than that of close (proximal) ones. However, mechanisms have been shown to equalize the contribution of such synapses, which resulted in the concept of “democracy of synapses” (Clifton C. Rumsey, LF Abbott, 2006). Aligning the contribution of synapses along a dendrite branch allows us to consider branches as independent logical elements whose signals are somehow processed by a neuron. This means that theoretically, by changing the configuration of the dendrite and the response of the soma, it is possible to obtain neurons with different logical properties.
For example, in the popular concept of “hierarchical temporal memory” by Jeff Hawkins, neurons are used that use autonomous elements operating in the “or” mode (figure below).
Neuron model with a set of dendritic logical autonomous elements in comparison with a pyramidal neuron (Hawkins, 2011) It is appropriate to assume that the real brain also operates with neurons with a significant variety of properties.
We now turn, in fact, to the description of the associative event memory. Suppose we have two types of neurons that form a flat cortex. The receptive fields of these neurons cover a certain local area of their environment, where neurons of both types fall. Conditionally we will separate them into two layers, remembering the cross-distribution of bonds.
Let us assign neurons of the first type such that they propagate a wave of information identifier. The neurons of the second type will make it necessary to distribute exclusively waves of hippocampal identifiers (figure below).
Propagation of two independent waves on neurons with a different type of mediator To do this, we will dissolve their axons and extrasynaptic receptors by the used mediators (table below). Please note that synaptic receptors are not important for us to specify the wave propagation.
| Synapses | Outside synapses | Axon |
---|
Type 1 | | A | A |
Type 2 | | B | B |
Neurotransmitter characteristics of neurons. Receptor sensitivity and axon release With this task of mediators and receptors, waves on two layers will not affect each other at all.
Now let us complicate neurons of the second type, we will make their dendritic trees consisting of two types of branches, differing in extrasynaptic mediators. In modeling, homogeneous branches can be merged and, as a result, two dendritic segments operating according to the “or” principle can be left (table below).
| Segment | Synapses | Outside synapses | Axon |
---|
Type 1 | | | A | A |
Type 2 | one | | B | B |
| 2 | | A | |
Neurotransmitter characteristics of neurons. Receptor sensitivity and axon release. The neurons of the second type have two dendritic segments with receptors that are sensitive to mediators of different types Suppose that while neurons were learning how to propagate identifier waves, the second segment of the neurons of the second type, which is sensitive to the “alien” mediator, was blocked. All information necessary for the formation of waves in neurons of the second type will be deposited on the extra-synaptic memory of the first segment.
If we now include the second segments, then they will begin to memorize wave patterns propagating through neurons of the first type. We will assume that extra-synaptic memorization occurs at the moment when the neuron generates a spike. At the same time, not an instantaneous picture of activity at synapses is fixed, but accumulated over the entire beat of the wave propagation. The accumulation will allow us to memorize a fragment of the first level wave, even if the phases of the waves of two levels in the memorizing place do not coincide. That is, when the neuron of the second level gives out a spike, it will record a picture of information activity, even if it was some time ago.
Suppose we spent one measure of such memorization. All neurons of the second level, which propagate the hippocampal identifier wave, which are active in this cycle, will memorize in their second segments fragments of the information wave transmitted through neurons of the first type. This means that if we ever repeat the same wave of description, all the second-level neurons that recognize this picture will give a spike. The overall picture of these spikes will reproduce the same identifier of the hippocampus that was at the time of memorizing this information image.
So you can memorize various descriptive pictures. By repeating the corresponding descriptions, neurons of the second level will produce a total combination of all hippocampal identifiers that fall under the current description.
It can be seen that such memorization is extremely wasteful. We memorize each image on all active neurons of the second type. Although to reproduce the identifier, we need only a small local area that can trigger a wave. To remedy the situation, we will memorize the information wave not on all active neurons of the second type, but only on those that fall into areas with high activity caused by neurons of the first type. That is, recall that information is dualistic. It is both an identification wave and the activity patterns of the induced activity. The wave will give us a pattern to memorize, and patterns will indicate places for this.
The easiest way to choose a place is to use the same field of activity that we used for spatial self-organization of patterns. In this configuration, memorization can be represented as follows. In places of the cortex, where the information picture creates patterns of induced activity, a field of activity is formed. All active neurons of the second type, which propagate the hippocampal wave, for which the activity field is above a certain threshold, are fixed on the extra-synaptic memory of the picture described by the information wave.
By submitting the information wave request, we get the activity of the neurons of the second type, who recognized this request. Local groups of such neurons will emit hippocampal identifiers related to all memories associated with this query.
We showed how unique identifiers of memories can be fixed and restored. Earlier, we showed that if neuron detectors store the memory of such unique identifiers, then we can restore the images corresponding to the memorized moments. The table below shows the possible configuration of mediators for such memory.
| Segment | Synapses | Outside synapses | Axon |
---|
Type 1 | one | | A | A |
| 2 | | B | |
Type 2 | one | | B | B |
| 2 | | A | |
Neurotransmitter characteristics of neurons. Receptor sensitivity and axon release. Biconnected configuration Setting the memorization area through the activity field is a rather rude tool. There are quite obvious ways to improve the design. For example, it is possible through synaptic training of neurons of the second type to create from them areas corresponding to the concepts located in this place of the cortex. Using the concept of a dendritic coincidence detector, it can be made so that the activity of a concept will be a prerequisite for both recording and reverse generating identifiers of memories associated with this concept.
In general, the wealth of tools provided by the wave model of the cortex with multisectional neurons makes it possible to implement fairly complex and interesting designs. We confine ourselves to the already simplest diagrams, so as not to completely confuse the narration.
Comments
To leave a comment
Logic of thinking
Terms: Logic of thinking